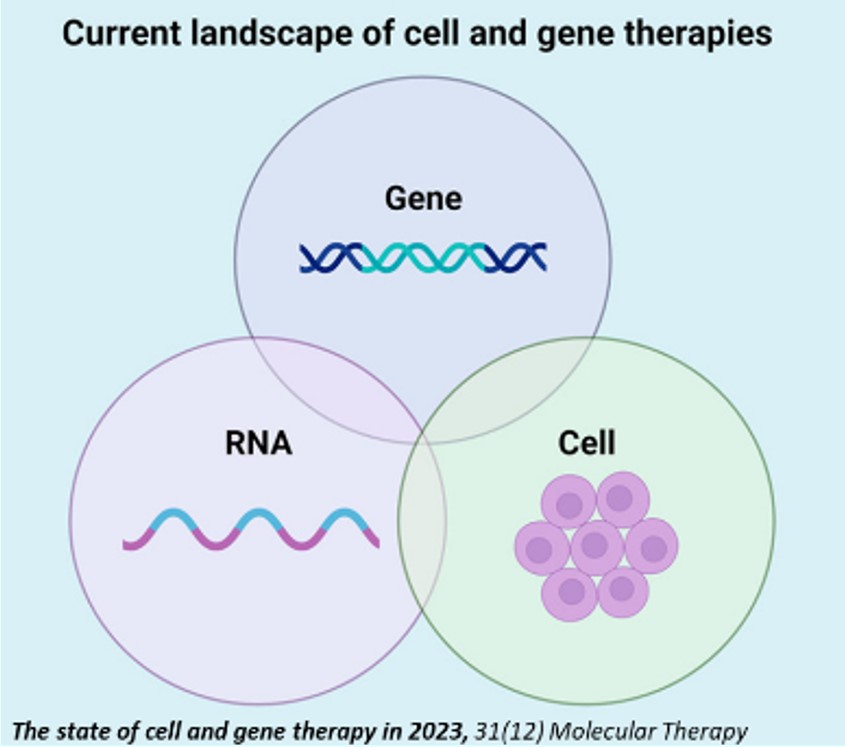
Biomanufacturing tools providers play a vital role by developing novel technologies and products to drive down manufacturing costs. This blog discusses 3 areas of technology powering the future of cell and gene therapies (CGT) manufacturing with design and development insights for each area: decentralized manufacturing, closed & automated systems and single-use technologies.
Cell and gene therapies hold the potential to prevent and even cure a wide variety of diseases. With several headline-grabbing regulatory approvals in 2023, and significantly more expected in 2024, manufacturing challenges may hinder the journey to the market for these products. As an example, FDA director Peter Marks (Center for Biologics Evaluation & Research) recently stated that current manufacturing platforms for gene therapy production are too costly which limits availability. Check out my blog on BioProcessing 4.0: Building the Future of Bioprocessing for more details.
Decentralized Manufacturing
While centralized manufacturing is the most common production model for most biologics today, decentralized manufacturing is gaining traction due to the emergence of cell and gene therapy products. For autologous cell therapies (e.g. CAR T-cell therapies), where a patient’s blood is collected, then modified and returned to the patient for reinfusion, a centralized manufacturing model is laborious and time-consuming. Here, a decentralized manufacturing model can significantly streamline the cell therapy production process to reduce costs and improve access to care.
There are several challenges related to establishing a viable decentralized manufacturing process, including: production process complexity, substantial infrastructure requirements, stringent quality control, and the need for significant new capital investment. To overcome these challenges, advances in manufacturing technology are required such as the development of innovative, closed and automated manufacturing systems. These systems enable the performance of standardized CGT production processes with reduced infrastructure complexity and cost and improved quality due to reduced operator intervention through the integration of single-use components, process control systems, aseptic connectors and automation. Accordingly, there is currently intensive technology development focused on all these areas.
The advent of closed, automated systems for decentralized manufacturing networks has raised the bar for equipment developers. It brings a whole host of new requirements, not the least of which is much greater usability. Thus, employing experienced industrial designers and user experience experts can pay big dividends for both the equipment developer and the end-user.
Closed & Automated Systems
Cell and gene therapy production has historically been achieved using relatively complex and highly manual processes. These manual processes require highly skilled scientists physically implementing each step, making them prone to human error or contamination. Closing the workflow to eliminate manual interventions decreases the likelihood of human error and automation can then be leveraged to further drive efficiency and help reduce costs. Accordingly, a number of innovative groups and companies are developing automated cell and gene therapy production platform systems that promise to reduce manufacturing cost, reduce facility infrastructure requirements and improve product quality. While great strides have been made here, existing technical challenges in this area include: improved sensor designs and associated process analytics, development of intuitive user interfaces to enable lower skilled operators, a greater selection of aseptic connection/disconnection solutions, effective tools to validate “proof of system closure” prior to use, robust predictive models to ensure effective process control and suitable single-use, sterilizable system components for the entire manufacturing workflow.
Converting open workflows to closed systems can provide some specific challenges that can trip up the development pathway. For example, single-use system components may not be readily available for all workflow unit operations, meaning custom solutions may need to be developed. Also, proof of system closure (or integrity) is often overlooked as a key product requirement that can be challenging to carry for systems with a large number of components. For these reasons, it is best to work with knowledgeable partners to avoid time-consuming and costly missteps.
Single-use Technologies
Single-use technologies for biomanufacturing are defined as disposable products that are intended for one-time use, made of various types of medical-grade (Class VI) sterilizable plastics. They have the following advantages over reusable systems:
- Reduced contamination risk – systems may be pre-sterilized.
- Lower cost – eliminates the need for labor-intensive cleaning & sterilization as well a high capital facility investment.
- Reduced labor – when used in combination with automated systems.
- Enhanced flexibility – enable rapid changeovers and adapting to diverse product needs.
- Environmental sustainability – reduce water and energy consumption.
- Quality assurance – manufacturing process control provides consistent product quality.
Unlike fixed systems in traditional facilities, single-use systems can be more easily adapted to various production scales, from small batches for rare disease indications to large-scale for large patient populations. Thus single-use technologies can provide a ready solution for the evolving needs of the CGT market.
Effective closed, presterilized single-use systems for CGT manufacturing require the integration of many individual components that must meet stringent functional and quality standards. These include many new products introduced to the market in recent years, including bioreactors, pump-heads, valves, connectors, sensors, filters, storage/freezing bags, chromatography devices and cell separation devices. However, the evolving nature of the cell and gene therapy product landscape means that the tools needed to manufacture these will continue to evolve in the near-term. Current areas of development include improved single-use sensors, cell separation devices and aseptic connectors/disconnectors/re-connectors.
Single-use component manufacturing should be carried out under controlled environmental conditions (typically an ISO7 cleanroom). In general, polymeric component parts are typically joined by thermal bonding processes like ultrasonic welding, RF welding and hot plate welding to avoid the introduction of any additional materials such as adhesives that may compromise product purity due to leaching during use. Component parts are usually produced via injection molding or extrusion to ensure high product consistency and reduce production costs. Increasingly, single-use components are being fabricated using radiation sterilization-compatible polymers to facilitate the production of pre-sterilized assemblies suitable for use in the closed, automated manufacturing systems ideally suited for CGT manufacturing.
It is important to carefully consider material properties fully when designing new single-use components since these will dictate compatibility with available manufacturing processes and post-production processes like sterilization. While a number of terminal sterilization processes are available, the most common ones involve exposure to some form of ionizing radiation. This is a key compatibility concern for single use component material selection.
Summary
The future of cell and gene therapies promises exciting breakthroughs in the treatment and possible cure for many previously untreatable diseases. However, this promise can only be realized with the continued evolution of innovative manufacturing systems that leverage a wide range of cutting-edge technologies. Please contact us at StarFish Medical if you have questions or need help with product development challenges in the CGT equipment space.
Gary Skarja is a Bio Services Program Manager at StarFish Medical. Over his 23-year career in the commercial life sciences sector, he has led the development of new medical materials & devices, regenerative medicine technologies and single-use biomanufacturing tools at companies ranging from pre-revenue start-ups to a multibillion dollar global organization. Gary holds a B.Eng. and M.Eng. in Chemical Engineering from McMaster University and a Ph.D. in Chemical Engineering from the University of Toronto.